Advanced Neutron Scattering Techniques
Category
Characterization Tools
Laboratory
National Institute of Standards and Technology (NIST) Center for Neutron Research
Capability Expert(s)
Craig Brown ([email protected]), Ryan Klein ([email protected])
Description
The NIST Center for Neutron Research (NCNR) provides advanced characterization support for HyMARC collaborators. Neutrons are particularly effective for studying hydrogen in materials because 1H and 2H scatter neutrons well. Techniques at the NCNR probe hydrogen in materials on length scales ranging from sub-Angstrom to centimeters, and dynamic time scales ranging from nanoseconds to seconds. A large temperature range (10 K to 1200 K) and in situ gas dosing capabilities are readily available. mK and variable field environments are also available. In general, ≈ 1 cc of sample is required at minimum. These techniques include:
- In situ gas-dosed powder neutron diffraction—determines crystal structure of gas dosed material, revealing atomic scale information about where hydrogen is in the sample. See references 1 and 2. Samples must be crystalline.
- In situ gas-dosed inelastic neutron scattering—provides information on phonon density of states, especially sensitive to 1H, useful for studying chemical bonding and sorption in storage materials. See references 3 and 4. Samples can be crystalline or amorphous.
- In situ gas-dosed quasielastic neutron scattering—provides information about dynamic motion of 1H inside materials on the Ångstrom to 10’s of nanometers lengthscale and on dynamic timescales of 1 picosecond to 1 nanosecond. Samples can be crystalline or amorphous. See references 5–9.
- Small angle neutron scattering—analogous to SAXS measurements, SANS measurements provide structural information on the length scale of 10s to 100s of including information about pore size distribution, etc. Samples can be crystalline, amorphous, liquids, gels, etc. See reference 10.
- Prompt gamma-ray activation analysis—non-destructive elemental analysis for samples ranging between micrograms and grams. Samples can be of any form. See reference 11.
- Neutron reflectometry—probes chemical composition as a function of depth in films with sub-Ångstrom thickness resolution possible. See reference 12.
- Neutron imaging—provides information on the location of hydrogen in a sample (can be a full-scale device like a fuel cell) on the mm length scale and on the timescale of seconds in real time. See reference 13.)
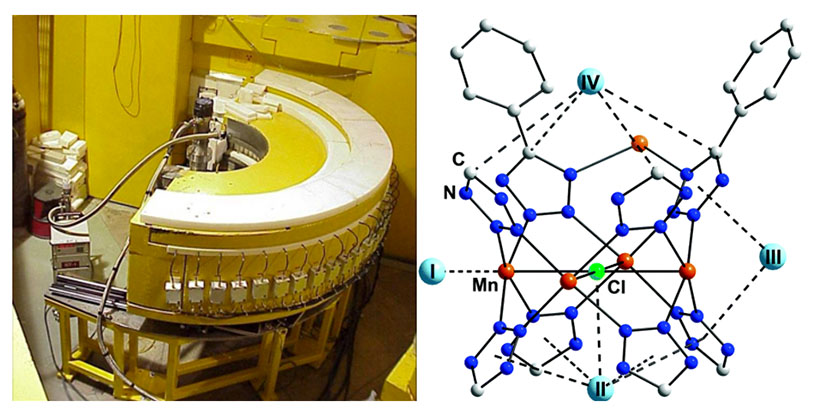
(Left) The high-resolution powder neutron diffractometer BT-1 at the NCNR enables in situ gas dosed measurements of candidate storage and carrier materials. (Right) Snippet of the crystal structure for D2-dosed Mn(btt) indicating primary hydrogen adsorption sites in a porous material, see reference 1.
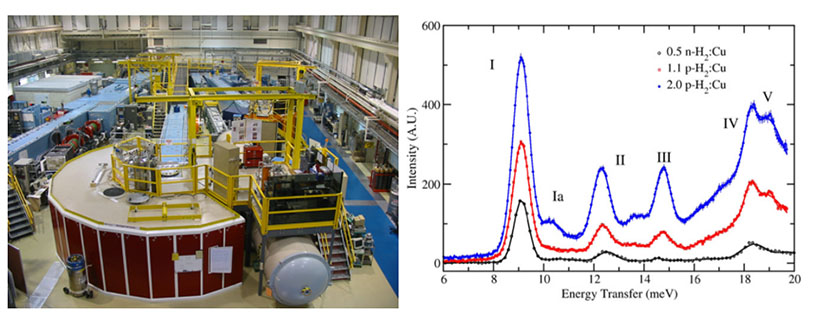
(Left) The disk chopper spectrometer (DCS) at the NCNR enables high-resolution QENS and INS measurements of in situ hydrogen gas dosed materials. (Right) INS data obtained for H2-dosed HKUST-1, see reference 4.
Status
Measurements currently available in collaboration with HyMARC. Please contact Craig or Ryan for more information.
References
- M. Dincǎ, A. Dailly, Y. Liu, C. M. Brown, D. A. Neumann, and J. R. Long "Gas adsorption microcalorimetry and modelling to characterize zeolites and related materials," J. Am. Chem. Soc. 128 (2005): 16876–16883. https://doi.org/10.1021/ja0656853.
- J. A. Mason, J. Oktawiec, M. K. Taylor, M. R. Hudson, J. Rodriguez, J. E. Bachman, M. I. Gonzalez, A. Cervellino, A. Guagliardi, C. M. Brown, P. L. Llewellyn, N. Masciocchi, and J. R. Long, "Methane storage in flexible metal–organic frameworks with intrinsic thermal management," Nature 527 (2015): 357–361. doi:10.1038/nature15732.
- C. Schönfeld, R. Hempelmann, D. Richter, T. Springer, A. J. Dianoux, J. J. Rush, T. J. Udovic, and S. M. Bennington “Dynamics of hydrogen in α-LaNi5 hydride investigated by neutron scattering,” Phys. Rev. B 50 (1994): 853–865. https://doi.org/10.1103/PhysRevB.50.853.
- C. M. Brown, Y. Liu, T. Yildirim, V. K. Peterson, and C. J. Kepert “Hydrogen adsorption in HKUST-1: a combined inelastic scattering and first principles study,” Nanotechnology 20 (2009): 1–11. DOI:10.1088/0957-4484/20/20/204025.
- C. Österberg, H. Fahlquist, U. Häussermann, C. M. Brown, T. J. Udovic, and M. Karlsson "Dynamics of Pyramidal SiH3–Ions in ASiH3 (A = K and Rb) Investigated with Quasielastic Neutron Scattering." J. Phys. Chem. C 120 (2016): 6369–6376. https://doi.org/10.1021/acs.jpcc.6b00363
- A.V. Skripov, O. Isnard, N.V. Mushnikov, P.B. Terent'ev, V.S. Gaviko, T.J. Udovic, “Hydrogen dynamics in the hexagonal Ho2Fe17H4 and Y2Fe17H4.2: Inelastic and quasielastic neutron scattering studies,” J. Alloys Compd. 720 (2017): 277–293. https://doi.org/10.1016/j.jallcom.2017.05.300.
- J. Purewal, J. B. Keith, C. C. Ahn, C. M. Brown, M. Tyagi, and B. Fultz. "Hydrogen diffusion in potassium intercalated graphite studied by quasielastic neutron scattering." J. Chem. Phys. 137, (2012): 224704. https://doi.org/10.1021/acs.jpcc.6b02431
- S. NaraseGowda, C. M. Brown, M. Tyagi, T. Jenkins, and T. A. Dobbins. "Quasi-elastic neutron scattering studies of hydrogen dynamics for nanoconfined NaAlH4." J. Phys. Chem. C 120 (2016): 14863-14873.
- M. Kofu, N. Hashimoto, H. Akiba, H. Kobayashi, H. Kitagawa, M. Tyagi, A. Faraone, J. R. D. Copley, W. Lohstroh, and O. Yamamuro. "Hydrogen diffusion in bulk and nanocrystalline palladium: A quasielastic neutron scattering study." Phys. Rev. B 94, no. 6 (2016): 064303.
- A. J. Allen, L. Espinal, W. Wong-Ng, W. L. Queen, C. M. Brown, S. R. Kline, K. L. Kauffman, J. T. Culp, C. Matranga “Flexible metal-organic framework compounds: In situ studies for selective CO2 capture,” J. Alloys Compd. 647 (2015): 24–34. https://doi.org/10.1016/j.jallcom.2015.05.148.
- 11. R. L. Paul, H. M. Privett III, R. M. Lindstrom, W. J. Richards, and R. R. Greenberg, "Determination of Hydrogen in Titanium Alloys by Cold Neutron Prompt Gamma Activation Analysis", Metall. Mater. Trans. A, 27A (1996): 3682-3687. https://doi.org/10.1007/BF02595460.
- D. Vogt, B. O'Brien, D. R. Allee, D. Loy, B. Akgun, S. K. Satija “Distribution of hydrogen in low temperature passivated amorphous silicon (a-Si:H) films from neutron reflectivity,” J. Non. Cryst. Solids 357 (2011): 1114–1117. https://doi.org/10.1016/j.jnoncrysol.2010.11.030.
- Park, Xianguo Li, D. Tran, T. Abdel-Baset, D.S. Hussey, D.L. Jacobson, M. Arif “Neutron imaging investigation of liquid water distribution in and the performance of a PEM fuel cell,” Int. J. Hydrog. Energy 33 (2008): 3373–3384. https://doi.org/10.1016/j.ijhydene.2008.03.019.
- A. Klein, H. A. Evans, B. A. Trump, T. J. Udovic, C. M. Brown, 10.02 - Neutron scattering studies of materials for hydrogen storage, in Comprehensive Inorganic Chemistry III, Ed. Jan Reedijk, Kenneth R. Poeppelmeier, Elsevier, 2023, 3–50. ISBN 9780128231531, https://doi.org/10.1016/B978-0-12-823144-9.00028-5.